Carl S. Thummel
Professor of Human Genetics
Developmental Gene Regulation
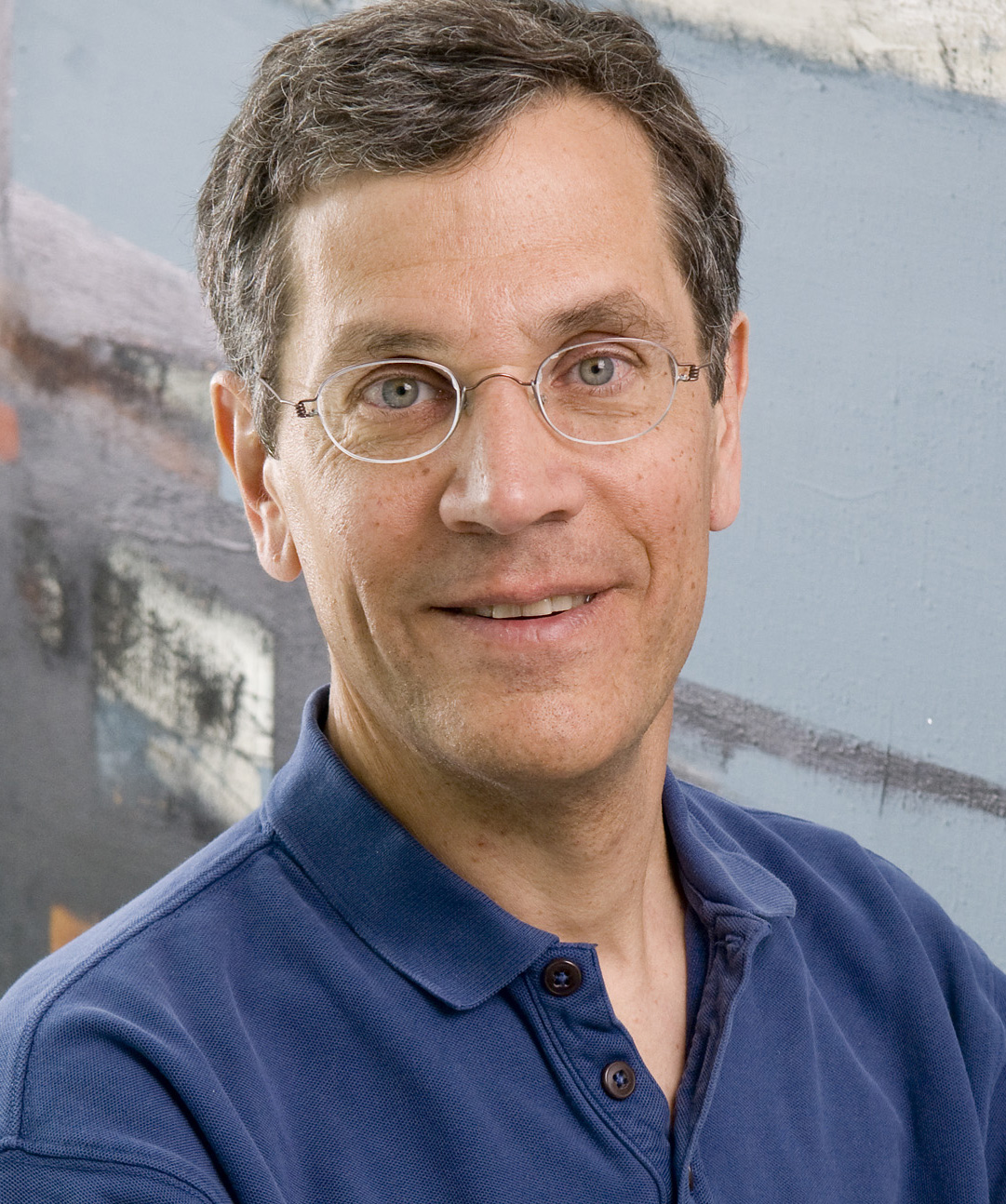
Molecular Biology Program
Education
B.A. Colgate University
Ph.D. University of California, Berkeley
Research
Our lab is studying the regulation of metabolism using the fruit fly, Drosophila melanogaster, as a model system. Remarkably, in spite of its small size and differences from mammals, many of the basic metabolic pathways are conserved through evolution from flies to humans. Flies have analogs of the basic tissues that control metabolism, including the equivalents of a liver, intestine, adipose tissue, pancreas, and kidneys. Many of the basic metabolic regulatory circuits are intact. Thus, for example, flies secrete insulin in response to elevated levels of circulating nutrients, and mobilize stored energy in response to a hormone related to glucagon. Flies are also subject to similar metabolic disorders as people, and thus can acquire type 2 diabetes if exposed to a high sugar diet, or can more than double their fat content and acquire heart disease when subjected to a high fat diet. They are also responsive to drugs used to treat metabolic disorders, such as the weight loss drug Alli (Orlistat). Our lab is studying the basic molecular mechanisms by which energy metabolism is controlled using the wide range of genetic tools available in Drosophila. We seek to uncover fundamental aspects of metabolic regulation that are conserved through evolution, with the aim of preventing and curing human disease. There are two major lines of study underway in the Thummel lab: (1) the transcriptional regulation of metabolism by nuclear receptors and (2) functions for evolutionarily conserved mitochondrial proteins.
Nuclear receptors are a family of ligand-regulated transcription factors that bind small lipophilic compounds, such as fatty acids, sterols and bile acids. In response to these signals, nuclear receptors regulate the expression of genes in specific metabolic pathways. The Drosophila genome encodes 18 nuclear receptors, compared to 48 in humans and 284 in C. elegans, providing the smallest complete set of these factors in any genetic model system. In spite of this small number, the fly nuclear receptors represent all major subclasses of human receptors, providing a good model for studying nuclear receptor regulation and function. Our current research in this area is focused on three nuclear receptors that play central roles in metabolism: dHNF4, E78, and dERR. Our goal is to extend our understanding of nuclear receptor signaling in new directions by using Drosophila as a genetic tool to define receptor regulation and function in the context of an intact animal, with an emphasis on characterizing those pathways that are held in common with their mammalian homologs. We anticipate that, as with past studies in Drosophila, discoveries stemming from our work will improve our design of mouse models for future functional studies and provide new directions for combating critical human diseases associated with nuclear receptor dysfunction, including cardiovascular disease, diabetes, and obesity.
Mitochondria are dynamic and complex organelles that play a central role in all aspects of biology, including energy production, intermediary metabolism, and apoptosis. In addition, mitochondrial dysfunction is associated with a wide range of diseases, including cancer, type 2 diabetes, and neurodegenerative disorders. As a result of these critical activities, many efforts have focused on defining the mitochondrial proteome, with over 1,000 proteins identified to date in mammals. Remarkably, however, one-fifth of these proteins remain largely uncharacterized. Our lab is studying the functions of key evolutionarily conserved mitochondrial proteins (MCPs) in close collaboration with Jared Rutter’s lab in the Biochemistry Department. We are conducting detailed characterization of MCP function in Drosophila, building off results obtained from analysis of the yeast homologs by the Rutter lab. Our goal in this research is to use Drosophila to provide new insights into the biological and physiological functions of these MCPs that can guide our understanding of how these proteins impact human health and disease.
References
-
Beebe, K, Robins, MM, Hernandez, EJ, Lam, G, Horner, MA, Thummel, CS (2020) Drosophila Estrogen-Related Receptor directs a transcriptional switch that supports adult glycolysis and lipogenesis. Genes & Dev., 34: 701-714.
-
Wisidagama, DR, Thummel, CS (2019) Regulation of Drosophila intestinal stem cell proliferation by enterocyte mitochondrial pyruvate metabolism. G3, Genes, Genomes, Genetics, 9: 3623-3630.
-
Marxreiter, S, Thummel, CS (2018) Adult functions for the Drosophila DHR78 nuclear receptor. Dev Dynamics, 247: 315–322.
-
Storelli, G, Nam, H-J, Simcox, J, Villaneuva, C, Thummel, CS (2019) Drosophila HNF4 directs a switch in lipid metabolism that supports the transition to adulthood. Dev. Cell, 48: 200-214.
-
Wisidagama, DR, Thomas, SM, Lam, G, Thummel, CS (2019) Functional analysis of Aarf Domain-Containing Kinase 1 in Drosophila melanogaster. Dev. Dynamics, doi: 10.1002/dvdy.66.
-
Praggastis, SA, Thummel, CS (2017) Right time, right place: the temporal regulation of developmental gene expression. Genes & Development, 31: 847-848.
-
Palu, RA, Praggastis, SA, Thummel, CS (2017) Parental obesity leads to metabolic changes in the F2 generation in Drosophila. Mol. Metab., 6: 631-639.
-
McCommis, KS, Hodges, WT, Bricker, DK, Wisidagama, DR, Compan, V, Remedi, MS, Thummel, CS, Finck, BN (2016) An ancestral role for the Mitochondrial Pyruvate Carrier in glucose-stimulated insulin secretion. Mol. Metab., 5: 602-614.
-
Palu, RA, Thummel, CS (2016) Sir2 acts through Hepatocyte Nuclear Factor 4 to maintain insulin signaling and metabolic homeostasis in Drosophila. PLoS Genetics, 12: e1005978.
-
Barry, WE, Thummel, CS (2016) The Drosophila HNF4 nuclear receptor promotes glucose-stimulated insulin secretion and mitochondrial function in adults. eLife, DOI: 10.7554/eLife.11183.
-
Somer, R, Thummel, CS (2015) Linking nutrients to growth through a positive feedback loop. Developmental Cell, 35: 265-266.
-
Somer, RA, Thummel, CS (2014) Epigenetic inheritance of metabolic state. Current Opinions in Genetics and Development, 27: 43-47.
-
Tennessen, JM, Barry, W, Cox, J, Thummel, CS (2014) Methods for studying metabolism in Drosophila. Methods, 68: 105-115.
-
Na, U, Yu, W, Cox, JE, Bricker, DK, Brockmann, K, Rutter, J, Thummel, CS, Winge, DS (2014) Two LYR assembly factors SDHAF1 and SDHAF3 mediate maturation of the iron-sulfur subunit of succinate dehydrogenase. Cell Metabolism, 20: 253-66.
-
Van Vranken, JG, Bricker, DK, Dephoure, N, Gygi, SP, Cox, JE, Thummel, CS, Rutter, J (2014) SHDAF4 promotes mitochondrial succinate dehydrogenase activity and prevents neurodegeneration. Cell Metabolism, 20: 241-52.
-
Tennessen, JM, Bertagnolli, NM, Evans, J, Sieber, MH, Cox, J, Thummel, CS (2014) Coordinated metabolic transitions during Drosophila embryogenesis and the onset of aerobic glycolysis. G3; Genes, Genomes, Genetics, 4: 839-850.
-
Bricker, DK, Taylor, EB, Schell, JC, Orsak, O, Boutron, A, Chen, Y-C, Cox, JE, Cardon, CM, VanVranken, J, Dephoure, N, Redin, C, Boudina, S, Gygi, SP, Brivet, M, Thummel, C, Rutter, J (2012) A Mitochondrial Pyruvate Carrier required for pyruvate uptake in yeast, Drosophila, and humans. Science, 337: 96-100.
-
Sieber, MH, Thummel, CS (2012) Coordination of triacylglycerol and cholesterol homeostasis by DHR96 and the Drosophila LipA homolog magro.Cell Metabolism, 15: 122-127.
-
Misra, J, Horner, MA, Lam, G, Thummel, CS (2011) Transcriptional regulation of xenobiotic detoxification in Drosophila. Genes & Development, 25: 1796-1806.
-
Seay, DJ, Thummel, CS (2011) The circadian clock, light, and cryptochrome regulate feeding and metabolism in Drosophila.Journal of Biological Rhythms, 26: 497-506.
-
Tennessen, JM, Thummel, CS (2011) Coordinating growth and maturation – insights from Drosophila. Current Biology, 21: R750-R757.
-
Tennessen, JM, Baker, KD, Lam, G, Evans, J, Thummel, CS (2011) The Drosophila Estrogen-Related Receptor directs a metabolic switch that supports developmental growth. Cell Metabolism, 13: 139-148.
-
Ruaud, A-F, Lam, G, Thummel, CS (2011) The Drosophila NR4A nuclear receptor DHR38 regulates carbohydrate metabolism and glycogen storage. Molecular Endocrinology, 25: 83-91.
-
Ruaud, A-F, Thummel, CS (2010) The Drosophila nuclear receptors DHR3 and βFTZ-F1 control overlapping developmental responses in late embryos. Development, 137: 123-131.
-
Sieber, M, Thummel, CS (2009) The DHR96 nuclear receptor controls triacylglycerol homeostasis in Drosophila. Cell Metabolism, 10: 481-490.
-
Horner, MA, Pardee, K, Liu, S, King-Jones, K, Lajoie, G, Edwards, A, Krause, HM, Thummel, CS (2009) The Drosophila DHR96 nuclear receptor binds cholesterol and regulates cholesterol homeostasis. Genes & Development, 23: 2711-2716.
-
Palanker, L, Tennessen, JM, Lam, G, Thummel, CS (2009) Drosophila HNF4 regulates lipid mobilization and b-oxidation. Cell Metabolism, 9: 228-239.
-
Baker, KD, Thummel, CS (2007) Diabetic larvae and obese flies – emerging studies of metabolism in Drosophila. Cell Metabolism, 6: 257-266.
-
Baker, KD, Beckstead, RB, Mangelsdorf, DJ, Thummel, CS (2007) Functional interactions between the Moses corepressor and DHR78 nuclear receptor regulate growth in Drosophila. Genes & Development, 21: 450-464.
-
Palanker, L, Necakov, AS, Sampson, HM, Ni, R, Hu, C, Thummel, CS, Krause, HM (2006) Dynamic regulation of Drosophila nuclear receptor activity in vivo. Development, 133: 3549-3562.
-
King-Jones, K, Horner, MA, Lam, G, Thummel, CS (2006) The DHR96 nuclear receptor regulates xenobiotic responses in Drosophila. Cell Metabolism, 4: 37-48.
-
King-Jones, K, Charles, J-P, Lam, G, Thummel, CS (2005) The ecdysone-induced DHR4 orphan nuclear receptor coordinates growth and maturation in Drosophila. Cell, 121: 773-784.
-
King-Jones, K, Thummel, CS (2005) Nuclear receptors – a perspective from Drosophila. Nature Reviews Genetics, 6: 311-323.
-
Beckstead, RB, Thummel, CS (2006) Indicted – C. elegans caught using steroids. Cell, 124: 1137-1140.
-
Yin, VP, Thummel, CS (2004) A balance between the diap1 death inhibitor and reaper and hid death inducers controls steroid-triggered cell death in Drosophila. Proc. Natl. Acad. Sci. USA, 101: 8022-8027.
-
Kozlova, T, Thummel, CS (2003) Essential roles for ecdysone signaling during Drosophila mid-embryonic development. Science, 301: 1911-1914.
-
Bialecki, M, Shilton, A, Fichtenberg, C, Segraves, WA, Thummel, CS (2002) Loss of the ecdysteroid-inducible E75A orphan nuclear receptor uncouples molting from metamorphosis in Drosophila. Developmental Biology, 3: 209-220.
-
Ward, RE, Evans, J, Thummel, CS (2003) Genetic modifier screens in Drosophila demonstrate a role for Rho1 signaling in ecdysone-triggered imaginal disc morphogenesis. Genetics, 165: 1397-1415.
-
Ward, RE, Reid, P, Bashirullah, A, D’Avino, PP, Thummel, CS (2003) GFP in living animals reveals dynamic developmental responses to ecdysone during Drosophila metamorphosis. Developmental Biology, 256: 389-402.
-
Thummel, CS (2001) Molecular mechanisms of developmental timing in C. elegans and Drosophila. Developmental Biology, 1: 453-465.
-
Gates, J, Thummel, CS (2000) An enhancer trap screen for ecdysone-inducible genes required for Drosophila adult leg morphogenesis. Genetics, 156: 1765-1776.
-
Lam, G, Thummel, CS (2000) Inducible expression of double-stranded RNA directs specific genetic interference in Drosophila. Current Biology, 10: 957-963.
-
Jiang, C, Lamblin, A-F, Steller, H, Thummel, CS (2000) A steroid-triggered transcriptional hierarchy controls salivary gland cell death during Drosophila metamorphosis. Molecular Cell, 5: 445-455.